Lab cryostats for physics and material research
For decades, Janis has been the lab cryostat manufacturer of choice for researchers and engineers who require reliable, highly precise low-temperature cooling. In support of the worldwide physics and material research community, Lake Shore offers a wide range of Environment by Janis laboratory cryostats, including:
- LHe and LN2 cryogen lab cryostats
- Cryogen-free models, as well as a recirculating gas cooler (RGC) option for saving on LHe usage
- Ultra-high-vacuum (UHV) models—ideal for trapped ion studies or cold atom quantum simulation experiments
- Optical access models with window sets for measurement from UV to IR
- Low-vibration models optimized for microscopy applications, including those requiring short working distance, high spatial resolution, and photoluminescence measurements
- Models highly suited for Raman, NMR, and FTIR spectroscopy
- Designs available for ARPES, DLTS, and ESR spectroscopy integration
- <2 K base temperatures available
Sample environment | ||||||||||||||||||||
Sample type = all (non-conductive, conductive, solid, liquid, powder) | ||||||||||||||||||||
Sample type = only conductive, solid | ||||||||||||||||||||
Rotatable sample | ||||||||||||||||||||
Maximum temperature >325 K | ||||||||||||||||||||
Fast and easy sample change <10 min | ||||||||||||||||||||
Low vibration 40 nm | ||||||||||||||||||||
UHV | ||||||||||||||||||||
Typical applications | ||||||||||||||||||||
Mössbauer | ||||||||||||||||||||
Optoelectronics | ||||||||||||||||||||
Magnetotransport | ||||||||||||||||||||
Noble gas trapping | ||||||||||||||||||||
Electrical transport | ||||||||||||||||||||
Quantum computing | ||||||||||||||||||||
CCS-100 | ![]() | ✔ | ✔ | ✔ | ||||||||||||||||
CCS-300S | ✔ | ✔ | ✔ | ✔ | ||||||||||||||||
CCS-300ST | ✔ | ✔ | ✔ | |||||||||||||||||
CCS-400 | ✔ | ✔ | ✔ | ✔ | ||||||||||||||||
CCS-400H | ✔ | ✔ | ✔ | ✔ | ||||||||||||||||
CCS-XG | ✔ | ✔ | ✔ | ✔ | ||||||||||||||||
CCS-XG-UHV | ✔ | ✔ | ✔ | ✔ | ||||||||||||||||
CCS-TRAP | ✔ | ✔ | ||||||||||||||||||
CCS-800 | ![]() | ✔ | ✔ | ✔ | ✔ | |||||||||||||||
CCS-900 | ✔ | ✔ | ✔ | ✔ | ✔ | ✔ | ||||||||||||||
CCS-900T | ✔ | ✔ | ✔ | ✔ | ✔ | |||||||||||||||
SHI-950-LT | ✔ | ✔ | ✔ | ✔ | ✔ | ✔ | ||||||||||||||
SHI-950T-LT | ✔ | ✔ | ✔ | ✔ | ✔ | ✔ |
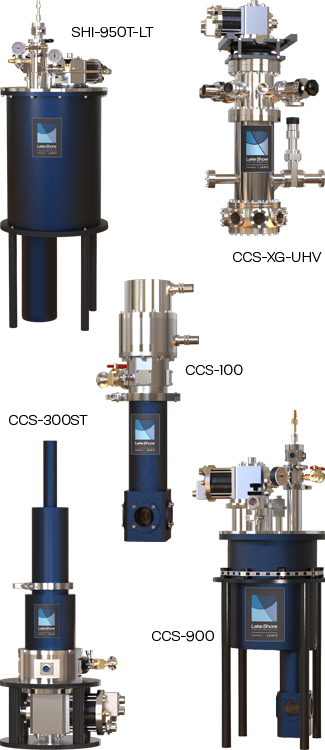
Sample environment | ||||||||||||||||||||
Sample type = non-conductive, conductive, solid, liquid, powder | ||||||||||||||||||||
Sample type = conductive, solid | ||||||||||||||||||||
Rotatable sample | ||||||||||||||||||||
Maximum temperature >325 K | ||||||||||||||||||||
LN2-cooled | ||||||||||||||||||||
LHe-cooled | ||||||||||||||||||||
Fast and easy sample change <10 min | ||||||||||||||||||||
Low vibration 15 nm | ||||||||||||||||||||
UHV | ||||||||||||||||||||
Typical applications | ||||||||||||||||||||
High Tc of superconducting materials | ||||||||||||||||||||
Optoelectronics | ||||||||||||||||||||
Magnetotransport | ||||||||||||||||||||
Microscopy | ||||||||||||||||||||
Raman | ||||||||||||||||||||
Electrical transport | ||||||||||||||||||||
Beamline | ||||||||||||||||||||
NMR | ||||||||||||||||||||
FTIR | ||||||||||||||||||||
STVP-100 | ![]() | ✔ | ✔ | ✔ | ✔ | ✔ | ✔ | ✔ | ✔ | |||||||||||
STVP-100-TH | ✔ | ✔ | ✔ | ✔ | ✔ | ✔ | ✔ | ✔ | ||||||||||||
STVP-NMR | ✔ | ✔ | ✔ | ✔ | ✔ | ✔ | ||||||||||||||
STVP-FTIR | ✔ | ✔ | ✔ | ✔ | ✔ | ✔ | ✔ | |||||||||||||
VNF-100 | ✔ | ✔ | ✔ | ✔ | ✔ | ✔ | ✔ | |||||||||||||
VNF-100-TH | ✔ | ✔ | ✔ | ✔ | ✔ | ✔ | ✔ | ✔ | ||||||||||||
ST-100 | ![]() | ✔ | ✔ | ✔ | ✔ | ✔ | ✔ | ✔ | ||||||||||||
ST-100-H | ✔ | ✔ | ✔ | ✔ | ✔ | ✔ | ✔ | |||||||||||||
ST-300 | ✔ | ✔ | ✔ | ✔ | ✔ | ✔ | ✔ | ✔ | ||||||||||||
ST-300-C | ✔ | ✔ | ✔ | ✔ | ✔ | ✔ | ✔ | ✔ | ✔ | |||||||||||
ST-400 | ✔ | ✔ | ✔ | ✔ | ✔ | ✔ | ||||||||||||||
ST-400-H | ✔ | ✔ | ✔ | ✔ | ✔ | ✔ | ||||||||||||||
ST-500 | ✔ | ✔ | ✔ | ✔ | ✔ | ✔ | ✔ | ✔ | ✔ | |||||||||||
ST-500-C | ✔ | ✔ | ✔ | ✔ | ✔ | ✔ | ✔ | ✔ | ✔ | |||||||||||
ST-FTIR | ✔ | ✔ | ✔ | ✔ | ✔ | ✔ | ✔ | |||||||||||||
VPF-100 | ✔ | ✔ | ✔ | ✔ | ✔ | ✔ | ||||||||||||||
VPF-100-H | ✔ | ✔ | ✔ | ✔ | ✔ | ✔ | ||||||||||||||
VPF-FTIR | ✔ | ✔ | ✔ | ✔ | ✔ | ✔ |
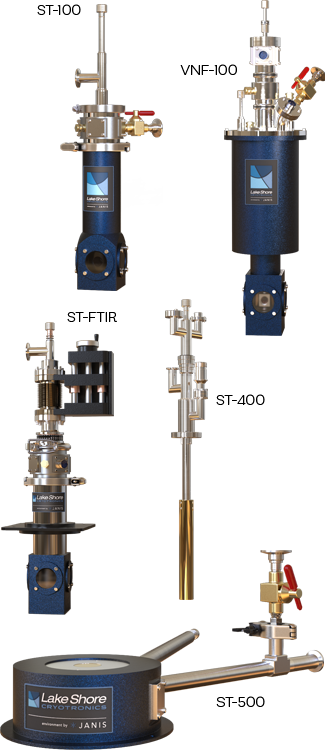
Typical laboratory cryostat applications
Magnetotransport studies materials in the presence of a magnetic field. The transport properties of charge carriers, such as their mobility and concentration, can be investigated using techniques such as magnetoresistance and Hall measurements.
Fourier transform infrared (FTIR) spectroscopy is used to study the vibrational modes of molecules. Infrared radiation excites the sample molecules. The absorption of the radiation by the sample is then measured, and the resulting spectrum can be used to identify the molecular bonds and functional groups present in the sample. Cryogenic FTIR spectroscopy is often used to study semiconductor materials.
Microscopy is using microscopes to observe and study small objects and structures. Cryogenic microscopy techniques are used to study a wide range of materials, including biological samples, polymers, semiconductors, and superconductors.
Cryostats specifically designed to fit within the space restrictions and mechanical constraints imposed by a physics accelerator beamline provide vibration-free operation and maximal thermal stability.
Noble gas trapping refers to the adsorption of noble gases, including helium, neon, argon, krypton, and xenon. The extraction of such gases from geological materials collected from volcanic hot springs provides insight into the planetary evolution of the Earth.
Electrical transport is a technique used to study the electrical properties of materials. This can include measurements of electrical resistance, conductivity, and other properties that are important for understanding the behavior of materials in various electronic and magnetic devices.
Optoelectronics studies electronic devices that interact with light, such as light-emitting diodes (LEDs), solar cells, and photodetectors. These optical components are cooled to reduce thermal noise and increase sensitivity to observe the components’ interaction between light and electrical signals. This is especially important in low-light applications, where even small amounts of noise can affect the accuracy of the measurements.
Nuclear magnetic resonance (NMR) spectroscopy can determine the sample’s atomic-level molecular structure. By analyzing the response of atomic nuclei to a strong magnetic field, NMR spectroscopy can provide information about the chemical environment and interactions of the atoms in the sample. NMR spectroscopy is particularly useful for studying the structure of molecules in solution, and it can also provide information on phase changes, conformational alterations, solubility, and diffusion potential.
Mössbauer spectroscopy studies low-energy gamma rays emitted and absorbed to understand the properties of a solid materials nuclear structure. A Mössbauer spectrometer attaches to the cryostat to excite and take measurements of the material being studied.
Some approaches to quantum computing require cryogenic temperatures. A UHV sample environment is needed for ion trap devices, as is extremely low vibration. With other approaches, fast sample exchange is useful for pre-screening devices.
The critical temperature (Tc) of superconducting materials is the temperature at which a material makes the transition from the normal to superconducting state. A popular characterization method is performing a 4-point resistivity measurement as a function of temperature to find out where the superconducting transition occurs. High Tc materials are ceramics that tend to be poor thermal conductors.
Raman spectroscopy is used to study the vibrational modes of molecules. A laser excites the sample molecules, causing them to vibrate. The scattered light from the sample is then analyzed to determine the frequencies of the molecular vibrations, which can provide information on the chemical composition and structure of the sample. Raman spectroscopy is often used to study inorganic and organic materials, and it is particularly useful for analyzing materials with complex structures, such as polymers.
FREE DOWNLOAD
How to choose the right research cryostat
A comprehensive overview of the critical factors to consider when selecting a cryostat
Get the guide